The year 2011 marks the 10th anniversary of an important advance in ultrafast laser pulses: For the first time, laser physicists were able to break the femtosecond (1 fs = 10-15 s) barrier to create attosecond (1 as = 10-18 s) pulses – the shortest light pulses ever generated in the laboratory.
Marie Freebody, Contributing Editor, marie.freebody@photonics.com
Attosecond light pulses are a technology generated by electrons, for electrons. By harnessing these ultrashort pulses, we can “see” electron motion in real time – which, if you are in the business of developing high-speed electronic components, is a valuable insight.
Electrons hold matter together – they determine the properties of the matter and the path of chemistry and biology – and keeping track of them requires dedicated optical elements. Observing the movement of electrons is also useful for developing solar cells as well as for understanding energy transportation in biological systems.

A view into the experimental chamber of the attosecond beamline AS-2: Atomic gas streams from a nozzle into the interaction volume, where attosecond XUV pulses and few-cycle laser pulses are employed for pump probe experiments. Photoelectrons are detected by a time-of-flight detector mounted from the top, and the ionized atoms are investigated by a mass spectrometer (reflectron) oriented horizontally. Courtesy of Thorsten Naeser, Max Planck Institute of Quantum Optics.
As we look deeper and deeper inside matter, not only do the dimensions get smaller, but also the timescale on which processes occur gets shorter. While femtosecond pulses are fast enough to probe molecular systems, observing the motion of electrons on the length and timescales of atoms requires laser pulses in the attosecond range.
The first single, isolated pulses lasting for less than 1 fs (measured at 650 as) were produced in 2001 by Dr. Reinhard Kienberger and professor Ferenc Krausz and his group at the Vienna University of Technology in Austria. Today, Krausz is director of the Max Planck Institute of Quantum Optics and professor at Ludwig Maximilian University, both in Munich, Germany.
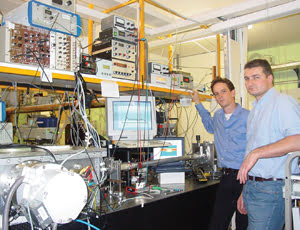
Right: After creating and measuring the first attosecond pulses (650 as in duration) in their Vienna laboratory in October 2001, Dr. Reinhard Kienberger and Dr. Michael Hentschel stand with the computer screen that displays the result. Courtesy of Ferenc Krausz.
“Attosecond flashes of light open the door for freezing the motion of electrons in atomic systems and thereby reconstructing this never-before-accessed motion from a series of freeze-frame snaps in the same way as femtosecond spectroscopy (based on femtosecond laser pulses) has permitted tracking the motion of atoms in molecular and solid-state systems,” Krausz said.
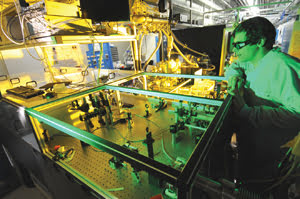
Left: At the Max Planck Institute of Quantum Optics in Germany, scientist Frederik Süßmann is working on the AS-5, an interferometric attosecond beamline for ultrahigh-vacuum experiments. Courtesy of Thorsten Naeser, Max Planck Institute of Quantum Optics.
Standing at the heart of attosecond pulse generation is re-collision, which was first put forward as a way of generating attosecond light pulses in 1993 by Paul Corkum of the Joint Attosecond Science Laboratory, University of Ottawa and National Research Council, Canada.
Corkum, who also proposed the attosecond streak camera, was co-author of the 2001 paper announcing the first attosecond pulses.
To perform the technique, known as high-order harmonic generation, a short infrared pulse is first fired at a cloud of gas atoms, typically those of a noble gas. The infrared pump pulse temporarily ionizes atoms in the gas, which releases an electron. When the electron re-collides with the nucleus from which it was separated, a short burst of radiation lasting a few hundred attoseconds is emitted.
Light generated in this way exhibits a much higher frequency than that of the pump pulse: typically in the extreme-ultraviolet (XUV) or soft-x-ray part of the spectrum. Controlled, reproducible generation of these x-ray pulses has been feasible by driving the process with infrared laser pulses consisting of a few well-controlled field oscillations. These waveform-controlled few-cycle pulses, together with the attosecond x-ray pulses synchronized to them, can be used to control and observe electron motion inside atoms.
In parallel to the triumph of attosecond pulse generation, attosecond electron pulse trains were generated via tunneling ionizing in atoms. Attosecond electron pulses have been used to probe dynamics in atomic and molecular systems via re-collision with the specimen from which they have been released.
“Re-collision can be thought of as an interferometer produced from an atom or molecule’s own electrons: Tunneling is the beamsplitter, and re-collision recombines the beams. Every one of the readers of Photonics Spectra will know how important an optical interferometer is. The electron interferometer will be equally important,” Corkum said.
“We can determine almost everything about an optical pulse with an interferometer. Therefore, with the electron interferometer underlying attosecond pulse generation, we can determine the characteristics of the re-collision electron, the orbital(s) from which the electron was extracted and any dynamics in the ionizing orbitals. We can also measure and control the attosecond pulses as they are being generated,” he added.
Currently, the minimum XUV pulse duration stands at 80 as; however, Corkum says that better results are yet to come. “Attosecond technology based on re-collision is the first optical technology that uses the highly nonlinear interaction of laser light with matter. Others will follow, leading us to still much shorter pulses,” he said. “I think that we have started on a long journey that will ultimately yield electron or photon pulses with duration below one attosecond. If so, not only the motion of electrons inside atoms but even motions inside atomic nuclei may become observable in real time.”
The field has made many dramatic advances since it was launched, and several groups have contributed their share. In 2010, Kienberger was awarded the International Commission for Optics’ prize for his work in attosecond science. The award highlights “outstanding scientific contributions to optics and photonics.” Kienberger is a professor of experimental physics at the Technical University of Munich, and he believes that groups working on attosecond technology are at the forefront of science.
“Since nobody entered this timescale until the beginning of the century, it is important that these groups learn how to handle pulses at this timescale, how to improve them and how to find ways to make them usable for applications,” he said. “Today, a vast number of groups are working with femtosecond lasers. In attosecond physics, however, the technology still has to be developed so that a broad range of scientists can use it.”
With the advent of waveform-controlled few-cycle laser pulses in 2003, attosecond scientists can now reliably produce as well as temporally characterize isolated attosecond pulses in the XUV. These novel ultrafast tools (isolated attosecond pulses and waveform-controlled few-cycle laser light) have meanwhile been used for a number of first-of-its-kind studies of atomic-scale electron motion.
One example has been the precise timing measurement of the photoemission process in atoms. The result, encountered in Krausz’s lab in 2010, was an unexpected revelation: a delay in the photo-effect. The explanation for the holdup is that different kinds of electrons leave their “mother” atom delayed by 20 as – the shortest interval ever measured.
Attosecond science: the mission
One of the great challenges for attosecond scientists is to step up photon energy from the current range of 30 to 130 eV to several hundred electron volts and – in the longer term – to the kilo-electronvolt range.
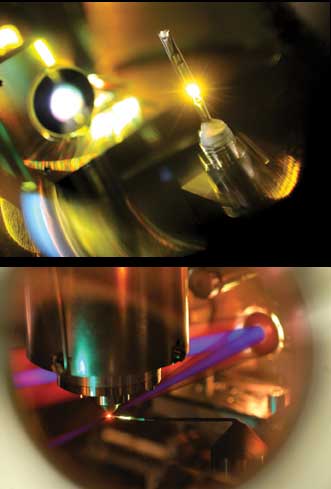
Top right: A nozzle emits a rare gas that converts laser pulses, coming from the right, into attosecond light flashes. Courtesy of Thorsten Naeser, Max Planck Institute of Quantum Optics.
Bottom right: Attosecond pulses of XUV light (depicted as a blue beam) are focused by a mirror (right) on a jet of neon atoms effusing from a thin valve. At the same time, an infrared beam is striking the atoms. Both beams in combination allow real-time observation of the motion of electrons in the neon atoms, as well as measurement of the duration of the attosecond pulse. Photo: Thorsten Naeser, Max Planck Institute of Quantum Optics; image editing: Christian Hackenberger, Ludwig Maximilian University of Munich.
These advances would greatly extend the scope of applications of attosecond technology from (the so-far realized) spectroscopy of electron dynamics in the valence and outer-core shells, to a wealth of processes happening within the inner shells. This is particularly relevant forthe development of x-ray lasers.
Krausz and colleagues are pursuing this goal by exploiting relativistic light-electron interactions with multiterawatt few-cycle light.
“Progressing further toward the 1-keV frontier will open the door to studying electronic phenomena in magnetic materials for the first time with attosecond resolution,” he said. “Once attosecond pulses at several kilo-electronvolt photon energy become available, they may make a long-standing dream of scientists come true: watching electron motion in real time with subatomic resolution in space and time via attosecond x-ray diffraction.”
Generating attosecond pulses at photon energies in the “water window” (270 to 550 eV) would open up many applications in biology. These would include probing coupled electronic-nuclear motion in organic molecular systems for the first time.
Electrons hold matter together – they determine the properties of the matter and the path of chemistry and biology – and keeping track of them requires dedicated optical elements. Observing the movement of electrons is also useful for developing solar cells as well as for understanding energy transportation in biological systems.

A view into the experimental chamber of the attosecond beamline AS-2: Atomic gas streams from a nozzle into the interaction volume, where attosecond XUV pulses and few-cycle laser pulses are employed for pump probe experiments. Photoelectrons are detected by a time-of-flight detector mounted from the top, and the ionized atoms are investigated by a mass spectrometer (reflectron) oriented horizontally. Courtesy of Thorsten Naeser, Max Planck Institute of Quantum Optics.
As we look deeper and deeper inside matter, not only do the dimensions get smaller, but also the timescale on which processes occur gets shorter. While femtosecond pulses are fast enough to probe molecular systems, observing the motion of electrons on the length and timescales of atoms requires laser pulses in the attosecond range.
The first single, isolated pulses lasting for less than 1 fs (measured at 650 as) were produced in 2001 by Dr. Reinhard Kienberger and professor Ferenc Krausz and his group at the Vienna University of Technology in Austria. Today, Krausz is director of the Max Planck Institute of Quantum Optics and professor at Ludwig Maximilian University, both in Munich, Germany.
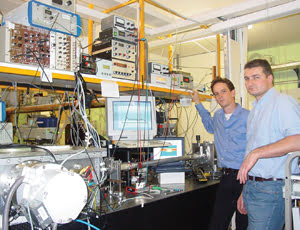
Right: After creating and measuring the first attosecond pulses (650 as in duration) in their Vienna laboratory in October 2001, Dr. Reinhard Kienberger and Dr. Michael Hentschel stand with the computer screen that displays the result. Courtesy of Ferenc Krausz.
“Attosecond flashes of light open the door for freezing the motion of electrons in atomic systems and thereby reconstructing this never-before-accessed motion from a series of freeze-frame snaps in the same way as femtosecond spectroscopy (based on femtosecond laser pulses) has permitted tracking the motion of atoms in molecular and solid-state systems,” Krausz said.
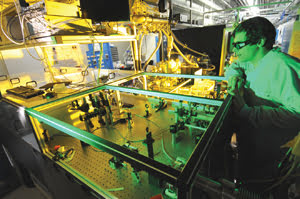
Left: At the Max Planck Institute of Quantum Optics in Germany, scientist Frederik Süßmann is working on the AS-5, an interferometric attosecond beamline for ultrahigh-vacuum experiments. Courtesy of Thorsten Naeser, Max Planck Institute of Quantum Optics.
Standing at the heart of attosecond pulse generation is re-collision, which was first put forward as a way of generating attosecond light pulses in 1993 by Paul Corkum of the Joint Attosecond Science Laboratory, University of Ottawa and National Research Council, Canada.
Corkum, who also proposed the attosecond streak camera, was co-author of the 2001 paper announcing the first attosecond pulses.
To perform the technique, known as high-order harmonic generation, a short infrared pulse is first fired at a cloud of gas atoms, typically those of a noble gas. The infrared pump pulse temporarily ionizes atoms in the gas, which releases an electron. When the electron re-collides with the nucleus from which it was separated, a short burst of radiation lasting a few hundred attoseconds is emitted.
Light generated in this way exhibits a much higher frequency than that of the pump pulse: typically in the extreme-ultraviolet (XUV) or soft-x-ray part of the spectrum. Controlled, reproducible generation of these x-ray pulses has been feasible by driving the process with infrared laser pulses consisting of a few well-controlled field oscillations. These waveform-controlled few-cycle pulses, together with the attosecond x-ray pulses synchronized to them, can be used to control and observe electron motion inside atoms.
In parallel to the triumph of attosecond pulse generation, attosecond electron pulse trains were generated via tunneling ionizing in atoms. Attosecond electron pulses have been used to probe dynamics in atomic and molecular systems via re-collision with the specimen from which they have been released.
“Re-collision can be thought of as an interferometer produced from an atom or molecule’s own electrons: Tunneling is the beamsplitter, and re-collision recombines the beams. Every one of the readers of Photonics Spectra will know how important an optical interferometer is. The electron interferometer will be equally important,” Corkum said.
“We can determine almost everything about an optical pulse with an interferometer. Therefore, with the electron interferometer underlying attosecond pulse generation, we can determine the characteristics of the re-collision electron, the orbital(s) from which the electron was extracted and any dynamics in the ionizing orbitals. We can also measure and control the attosecond pulses as they are being generated,” he added.
Currently, the minimum XUV pulse duration stands at 80 as; however, Corkum says that better results are yet to come. “Attosecond technology based on re-collision is the first optical technology that uses the highly nonlinear interaction of laser light with matter. Others will follow, leading us to still much shorter pulses,” he said. “I think that we have started on a long journey that will ultimately yield electron or photon pulses with duration below one attosecond. If so, not only the motion of electrons inside atoms but even motions inside atomic nuclei may become observable in real time.”
The field has made many dramatic advances since it was launched, and several groups have contributed their share. In 2010, Kienberger was awarded the International Commission for Optics’ prize for his work in attosecond science. The award highlights “outstanding scientific contributions to optics and photonics.” Kienberger is a professor of experimental physics at the Technical University of Munich, and he believes that groups working on attosecond technology are at the forefront of science.
“Since nobody entered this timescale until the beginning of the century, it is important that these groups learn how to handle pulses at this timescale, how to improve them and how to find ways to make them usable for applications,” he said. “Today, a vast number of groups are working with femtosecond lasers. In attosecond physics, however, the technology still has to be developed so that a broad range of scientists can use it.”
With the advent of waveform-controlled few-cycle laser pulses in 2003, attosecond scientists can now reliably produce as well as temporally characterize isolated attosecond pulses in the XUV. These novel ultrafast tools (isolated attosecond pulses and waveform-controlled few-cycle laser light) have meanwhile been used for a number of first-of-its-kind studies of atomic-scale electron motion.
One example has been the precise timing measurement of the photoemission process in atoms. The result, encountered in Krausz’s lab in 2010, was an unexpected revelation: a delay in the photo-effect. The explanation for the holdup is that different kinds of electrons leave their “mother” atom delayed by 20 as – the shortest interval ever measured.
Attosecond science: the mission
One of the great challenges for attosecond scientists is to step up photon energy from the current range of 30 to 130 eV to several hundred electron volts and – in the longer term – to the kilo-electronvolt range.
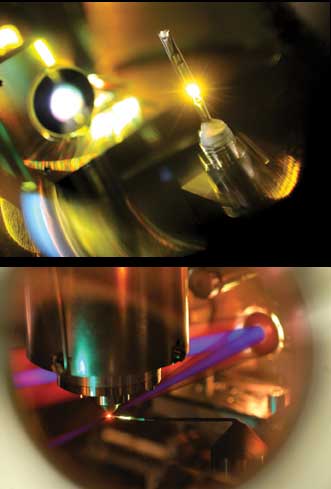
Top right: A nozzle emits a rare gas that converts laser pulses, coming from the right, into attosecond light flashes. Courtesy of Thorsten Naeser, Max Planck Institute of Quantum Optics.
Bottom right: Attosecond pulses of XUV light (depicted as a blue beam) are focused by a mirror (right) on a jet of neon atoms effusing from a thin valve. At the same time, an infrared beam is striking the atoms. Both beams in combination allow real-time observation of the motion of electrons in the neon atoms, as well as measurement of the duration of the attosecond pulse. Photo: Thorsten Naeser, Max Planck Institute of Quantum Optics; image editing: Christian Hackenberger, Ludwig Maximilian University of Munich.
These advances would greatly extend the scope of applications of attosecond technology from (the so-far realized) spectroscopy of electron dynamics in the valence and outer-core shells, to a wealth of processes happening within the inner shells. This is particularly relevant forthe development of x-ray lasers.
Krausz and colleagues are pursuing this goal by exploiting relativistic light-electron interactions with multiterawatt few-cycle light.
“Progressing further toward the 1-keV frontier will open the door to studying electronic phenomena in magnetic materials for the first time with attosecond resolution,” he said. “Once attosecond pulses at several kilo-electronvolt photon energy become available, they may make a long-standing dream of scientists come true: watching electron motion in real time with subatomic resolution in space and time via attosecond x-ray diffraction.”
Generating attosecond pulses at photon energies in the “water window” (270 to 550 eV) would open up many applications in biology. These would include probing coupled electronic-nuclear motion in organic molecular systems for the first time.
1 comment:
atto-seconds, like "atta-boys" don't last long
Post a Comment